International Workshop on Multi-messenger Tomography of the Earth
Amphitheater Pierre-Gilles de Gennes
APC-Université Paris Cité
The second Workshop on Multi-Messenger Tomography of the Earth will be held from the 4th to 7th of July, 2023 at Université Paris Cité in Paris (France), jointly organized by Laboratoire AstroParticule et Cosmologie and Institut de Physique du Globe de Paris.
Following up on the first edition held in 2022 (https://www.physics.utah.edu/mmte-2022/), the aim of the workshop is to bring together geoscientists and neutrino physicists to explore new pathways of combining their methodological approaches and data towards a multi-messenger tomography of the Earth. Sessions will include an overview of the methodologies for data inversion in both communities, and presentations of the theoretical and experimental state of the art in Earth core and mantle studies.
The last day of the workshop will be devoted to kickstarting the redaction of a White Paper on "Multi-messenger tomography of the Earth: science reach and methods".
We welcome abstracts from the community, and especially from early career scientists, for whom we hope to be able to provide some registration fee waivers. If you would like to request this support, please contact us by email.
It will be possible to follow the workshop remotely; however we strongly encourage the speakers to be present on site. Participants on-site are requested to pay a fee of 140 euro, covering the coffee breaks, a Wine and Cheese event and a Social Dinner (where accompanying persons are also welcome at an extra cost).
Zoom information for a remote participation:
https://u-paris.zoom.us/j/88633878822?pwd=VlljUGN5STdVL0g0aWhac2svcTBHZz09
ID meeting : 886 3387 8822
Password : 237398
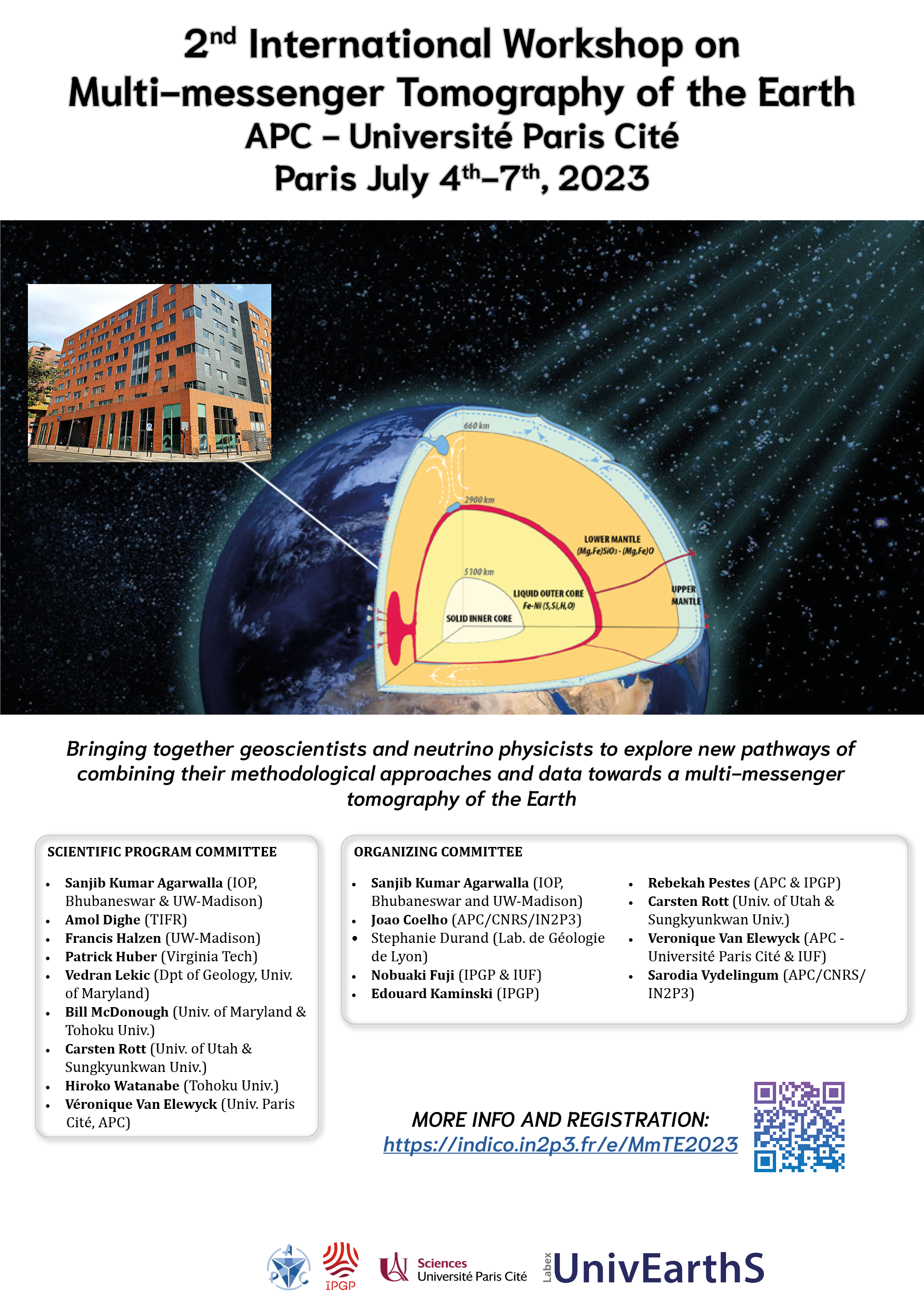
-
-
13:00
→
14:00
Registration 1h Amphitheater Pierre-Gilles de Gennes
Amphitheater Pierre-Gilles de Gennes
APC-Université Paris Cité
-
14:00
→
14:10
Welcome address on behalf of LabEx Univ'EarthS 10m Amphitheater Pierre-Gilles de Gennes
Amphitheater Pierre-Gilles de Gennes
APC-Université Paris Cité
Orateur: Nam Phan Van Song -
14:10
→
14:30
Introduction to the workshop 20m Amphitheater Pierre-Gilles de Gennes
Amphitheater Pierre-Gilles de Gennes
APC-Université Paris Cité
Orateurs: Nobuaki Fuji (IPGP), Véronique Van Elewyck (APC) -
14:30
→
15:50
Overview - neutrinos and geoscience Amphitheater Pierre-Gilles de Gennes
Amphitheater Pierre-Gilles de Gennes
APC-Université Paris Cité
Président de session: Véronique Van Elewyck (APC)-
14:30
Earth for neutrinos, neutrinos for Earth 40m
(REMOTE)
Not only does Earth produce neutrinos, it also provides a medium for neutrinos to pass through. This has already led to the discovery of neutrino oscillations, and hence of neutrino masses. Further, Earth affects the oscillations of neutrinos propagating through it, a phenomenon that has been instrumental for precision measurements of neutrino mixing parameters. Future experiments with neutrino beams and observations of atmospheric as well as astrophysical neutrinos will exploit Earth matter effects extensively to look for new interactions of neutrinos. On the other hand, neutrino experiments are sensitive to the electron densities in the Earth matter they pass through. Measurements of oscillations and attenuation of neutrinos passing through Earth would be able to provide independent information on the internal structure of the Earth, such as the chemical composition, the core mantle boundary, the density jumps, etc.
Orateur: Prof. Amol Dighe (Tata Institute of Fundamental Research (TIFR)) -
15:10
Geosciences for neutrino physicists: a primer 40m
Seismology uses energy from earthquakes and other seismic events to provide direct observations of Earth’s interior, and constraints on its physical parameters. Seismic data are sensitive to velocity and attenuation properties, as well as density, anisotropy, and scattering. These measurements are employed in combination with output from geodynamical simulations and mineral physics experiments and calculations to infer mantle temperature and composition. Subsequently, we are able to map mantle features such as discontinuities and scatterers, track convection patterns and flow stagnation, and identify regions associated with geochemical reservoirs.
In this talk I will present an overview of the use of seismology for constraining Earth’s mantle structure. I will summarise seismic data types and observations, introduce processing methodologies including tomography and coda-correlation, and review how seismology informs our understanding of mantle thermochemistry and dynamics via multidisciplinary approaches.
Orateur: Dr Lauren Waszek (James Cook University)
-
14:30
-
15:50
→
16:20
Coffee break 30m Amphitheater Pierre-Gilles de Gennes
Amphitheater Pierre-Gilles de Gennes
APC-Université Paris Cité
-
16:20
→
17:50
Overview - neutrinos and geoscience Amphitheater Pierre-Gilles de Gennes
Amphitheater Pierre-Gilles de Gennes
APC-Université Paris Cité
Président de session: Véronique Van Elewyck (APC)-
16:20
Structure and composition of the Earth 40m
The bulk silicate Earth’s (BSE) composition is the product of planetary accretion, core differentiation, and Moon formation. Given the BSE’s composition one can determine the composition of the bulk Earth and by subtraction calculate the core’s composition. The BSE represents the bulk Earth minus the core, which in today’s terms equals the modern mantle, the continental crust, and the hydrosphere-atmosphere systems. The modern mantle can be framed in terms of two compositionally distinct components, an enriched and a depleted mantle, with the latter as sampled by MORB (mid-ocean ridge basalts) and the former as sampled by OIBs (ocean island basalt).
The Earth’s surface heat flux is 46±3 TW (terrawatts, 1012 watts). Some fraction of this flux (~40% or ~20 TW) is derived from radioactive heat produced by the decay of U, Th, and K. Some 40% of the Earth’s budget of these elements is stored in the continental crust (~7.5 TW) and does not contribute to mantle heating. The remaining energy is primordial derived from accretion and core separation. The heat flux through the mantle also includes a core contribution (~10±5 TW; i.e., bottom heating of the mantle).
The rich record of seismic tomography documents ocean tectonic plates entering the mantle at oceanic trenches, plunging deep into the mantle, stagnating at the base of the Mantle Transition Zone (410-660 km depth), while others stagnating at ~1000 km depth, and others still plunge directly into the deep mantle. Collectively, these images reveal mass exchange between the upper and lower mantle and are consistent with whole mantle convection. The Mantle Transition Zone (MTZ) plays a major role in the differentiation of mantle. Mantle dynamics is controlled by its viscosity, which in turn is controlled by its water content and temperature. Evidence for core-mantle exchange remains elusive. Water is transported between the surface and interior, however, we are challenged to document if the net flux to the surface is positive or negative with time.
The Earth’s metallic core at ½ a radius and 1/3 of its mass, separated from the silicate Earth during planetary formation over a few 107 years, as documented by 182Hf-182W isotope system (t1/2 ~9 Ma). The core’s composition was established during this period and remained in isolation, because there is negligible evidence for any significant core-mantle mass exchange through time. The core is dominantly an FeNi alloy with about 10% of a light element component based on its density, as compared with an alloy at these PT conditions. The nature of this light element component is considerably discussed in the literature and not resolved. There is negligible evidence in support of radioactive elements in and heating the core.
Moon (1022 kg) formation happened anywhere between 50 Ma and 150 Ma after solar system initiation due to one or more impact events of Mars (1023 kg) sized impactors. The Moon’s chemistry indicates that the bulk of its composition is derived from the Earth’s mantle (1024 kg) and was unlikely to have involved core materials from either colliding body.
Orateur: Prof. Bill McDonough (University of Maryland) -
17:00
Current and future neutrino experiments with tomography potential 40m
(REMOTE)
Orateur: Prof. Carsten Rott (University of Utah/Sungkyunkwan University)
-
16:20
-
13:00
→
14:00
-
-
09:00
→
12:30
Methodologies and numerical approaches to inversion problems Amphitheater Pierre-Gilles de Gennes
Amphitheater Pierre-Gilles de Gennes
APC-Université Paris Cité
Président de session: Stephanie Durand (CNRS - LGLTPE)-
09:00
An Efficient Workflow for Global Seismic Tomography 30m
Understanding the Earth’s interior properties is vital for various research branches like geodynamics and plate tectonics and helps crucial estimations for seismic risk assessments and shake maps. Full seismic waveform inversion has emerged as a promising methodology to recover improved tomographic images of the mantle and crust. As the workflow solves the wave equation through numerical modelling, the resulting models are capable of accurately capturing and accounting for any observed wiggles in the seismic data. However, the computational demand of full-waveform inversion is a notorious drawback.
We present two approaches to reduce computational requirements for global tomography: dynamic mini-batches and wavefield-adaptive meshes. With dynamic mini-batches, only a subset of events is considered for each iteration, significantly reducing the overall number of required forward and adjoint simulations to compute model gradients. Wavefield-adaptive meshes minimize the number of elements in simulation meshes by designing the spectral-element meshes with a preferable geometry for each event. Applying this economic workflow to a global dataset of ~2000 events, we obtain a tomographic model explaining global waveform propagation for a minimum period of 35 s.
Building upon the previous concepts, we introduce the Collaborative Seismic Earth Model (CSEM), which embeds finely detailed regional tomographic models within a global context, resulting in a multi-scale representation of the Earth’s mantle and crust. In its current state, the model not only provides global waveform fits for minimum periods of 50 s but also surpasses this resolution down to 20 s in targeted regions.
Orateur: Dr Sebastian Noe (ETH Zurich) -
09:30
Computational and Statistical Aspects of Neutrino Oscillation Tomography 30mOrateur: Dr Joao Coelho (APC / CNRS)
-
10:00
An example of combined inversion: muon tomography 30m
Muon imaging has recently emerged as a powerful method to complement standard geophysical tools in the understanding of the Earth’s subsurface. Muon measurements yield a “radiography” of the average density along the muon path, allowing to image large volumes of a geological body from a single observation point. More traditional gravity field measurements are also sensitive to the near-surface density distribution and thus both methods care complementary. We will explore the joint inversion of muon data together with gravity data to estimate the three-dimensional density structure of the La Soufrière de Guadeloupe volcano. We discuss the advantages and challenges of such exercise and the resolution and potential improvements of the methods proposed.
Orateur: Dr Marina Rosas-Carbajal (IPGP) -
10:30
Coffe break 30m
-
11:00
3D density models from normal modes 30m
Normal modes, or whole Earth oscillations, are the only seismic data that are able to constrain density in the Earth in addition to velocity. Thus, normal modes are key for comparing and combining with constraints on density from neutrino data.
Normal modes are studied by using large earthquakes and Fourier transforming week-long seismograms from the time domain to the frequency domain. The normal mode spectra in a 1D spherical Earth model can be calculated exactly; for a given normal mode with angular order $l$ and overtone number $n$, the frequencies of the $2l+1$ eigenfunctions, or singlets, are degenerate. Adding ellipticity, rotation and 3D structure removes the degeneracy and results in splitting of the eigenfrequencies (similar to Zeeman splitting for the hydrogen atom). We use perturbation theory to calculate the splitting which also enables us to perform seismic tomography by comparing measured normal mode frequency splitting with calculations for 1D and 3D velocity and density models.
1D density models of the mantle, outer and inner core are made using the measured normal center frequencies. In these models we find, for example, that the outer core has a lightly larger density than PREM and that the inner core has a slightly lower density. Determining the precise density of the outer core and inner core is important because it determines the amount of light elements present in the core in addition to iron.
3D tomographic density models of the mantle are made using the measured singlet splitting of the normal modes, which we visually as maps showing how the normal mode center frequency varies regionally. Such images show regions with slow and fast velocity anomalies, including two large continental size regions just above the core mantle boundary, one located under the Pacific and the other one under Africa (the so called `LLSVPs'). These two regions have low shear wave velocity, but their role in mantle convection as either a thermal plume or a stable compositional pile is still heavily debated. Using our normal mode splitting measurements, we find that the two LLSVPs in the lower mantle are partially dense at their base while the remaining parts are light. Using a recently developed neural network, we interpret our model which shows that the dense and light parts both have a high temperature; the larger density comes from an increase in iron content which may be stable in mantle convection.
Orateur: Prof. Arwen Deuss (Utrecht University) -
11:30
Neutrino Tomography with 3D Earth Models 20m
(REMOTE)
Neutrinos can be used to study the interiors of various objects that are difficult to fully probe by classical means. In the case of the Earth, they provide complementary information to seismic waves because of the imprint of matter effects on their oscillations. This alternative approach may bring new insights on open questions regarding the composition, structure, and dynamics of the deep Earth. Though seismic wave data has been uncovering large asymmetric features inside the Earth, neutrino simulations have largely kept using a spherically symmetric Earth model. This is problematic if we want to use neutrinos to help disentangle various properties of matter in Large Low-Shear-Velocity Provinces (LLSVPs), for example. In this contribution, I will be talking about how to expand simulations from using this effectively 1D Earth model into using a full 3D Earth model, allowing for asymmetries inside the Earth. I will specifically focus on how we did that with OscProb, a C++ programming library for calculating neutrino oscillation probabilities.
Orateur: Rebekah Pestes (APC/IPGP) -
11:50
Round table on inversion methods and data combination 40mOrateurs: Dr Joao Coelho (APC / CNRS), Prof. Sanjib Kumar Agarwalla (Institute of Physics, Bhubneswar, India & University of Wisconsin-Madison, USA)
-
09:00
-
12:30
→
14:00
Lunch 1h 30m Amphitheater Pierre-Gilles de Gennes
Amphitheater Pierre-Gilles de Gennes
APC-Université Paris Cité
-
14:00
→
18:40
Open questions in the study of Earth's mantle and core Amphitheater Pierre-Gilles de Gennes
Amphitheater Pierre-Gilles de Gennes
APC-Université Paris Cité
Président de session: Nobuaki Fuji (IPGP)-
14:00
Large low-shear velocity provinces 25m
(REMOTE)
Orateur: Dr Laura Cobden (University of Utrecht) -
14:25
Mantle convection modeling 25m
I will give an overview over key aspects of geodynamic numerical modelling of the solid Earth following our review paper (van Zelst et al. 2022) and the annual EGU short course titled “Geodynamics 101: Numerical modelling”. Numerical modelling aims to gain scientific understanding of a certain real-Earth observation. It consists of a physical and numerical model, a numerical code, a specific model setup, in-depth model validation, production runs and their analysis, and finally the fair communication of findings and software and data management. The presentation will provide do’s and don’ts for any modeller, but also any non-modeller to better collaborate on and/or review numerical modelling research.
Orateur: Dr Fabio Crameri (Undertone.design) -
14:50
Perspectives for Atmospheric Neutrino Studies with Hyper-Kamiokande 25m
Hyper-Kamiokande is a next-generation neutrino experiment that is under construction in Japan. Among its various physics goals, it will be used to make long-baseline neutrino oscillation measurements. This will combine neutrinos from the J-PARC accelerator (which is now serving the T2K experiment) as well as neutrinos created by interactions of primary cosmic rays from the atmosphere. The Hyper-Kamiokande far detector design has a fiducial volume 8 times the size of the currently-running Super-Kamiokande detector and will be instrumented with new photosensors that offer significant improvements in performance. In this talk, we will focus specifically on how Hyper-K will be capable of probing CP-violation and the neutrino mass hierarchy with atmospheric neutrinos alone. A general overview of atmospheric neutrinos will be tied to the reconstruction/classification capabilities of Hyper-K and finally to its sensitivity over time to these two physics questions.
Orateur: Dr Andrew Santos -
15:15
JUNO's Prospect for atmospheric neutrinos 20m
The Jiangmen Underground Neutrino Observatory (JUNO) is a next-generation neutrino detector under construction in Guangdong, China. With a 20-kt target, JUNO will be the largest liquid scintillator (LS) detector ever built. JUNO’s instrumental setup includes nearly 18,000 20-inch photomultiplier tubes, providing an extensive 78% optical coverage.
The primary objective of JUNO is to determine the neutrino mass ordering (NMO) by studying the vacuum-dominated oscillation pattern of reactor antineutrinos. However, JUNO’s physics potential extends beyond reactor neutrinos thanks to its unique design. JUNO will be able to probe atmospheric neutrinos at lower energies than the current large water/ice Cherenkov detectors. This talk will provide current updates on various aspects of atmospheric neutrino research at JUNO, including a preliminary sensitivity study to NMO, energy and directionality reconstruction, neutrino interaction models in LS, and a primary neutrino energy spectrum based on a probabilistic unfolding method.
Orateur: Mariam Rifai (FZ Juelich and RWTH Aachen university) -
15:35
Coffee break 30m
-
16:05
Perspectives for atmospheric neutrino detection and Earth tomography with the KM3NeT detectors 25m
The KM3NeT Collaboration is incrementally building a network of water-Cherenkov neutrino observatories in the Mediterranean Sea, consisting of two telescopes, named ARCA and ORCA (respectively for Astroparticle and Oscillation Research with Cosmics in the Abyss), sharing the same detection technology. Owing to the different energy ranges covered by its two detectors, KM3NeT will perform both oscillation and absorption neutrino tomography using mainly atmospheric neutrinos. After describing the status of ORCA and ARCA and discussing the latest results obtained so far for atmospheric neutrinos with partial detector configurations, we will present preliminary sensitivity studies addressing the potential of the full KM3NeT detectors for neutrino tomography of the Earth.
Orateur: Prof. Véronique Van Elewyck (APC) -
16:30
Aspects of Neutrino Tomography of the Earth 25m
Aspects of the theory of neutrino oscillation
tomography of the Earth will be considered.
The sensitivity of the ORCA detector to deviations of the Earth
i) outer core (OC) density, ii) inner core (IC) density, iii) total core density, and iv)
mantle density, from their respective PREM densities will
be discussed.Orateur: Prof. Serguey Petcov (INFN/SISSA, Trieste, Italy) -
16:55
Probing the interior of Earth using neutrino oscillations in IceCube-DeepCore 35m
(REMOTE)
The information about the internal structure of Earth is obtained mainly using seismic studies and gravitational measurements. Neutrinos can be used as an independent probe to explore the interiors of Earth. While passing through Earth, the upward-going atmospheric neutrinos with multi-GeV energies experience matter effects due to the coherent forward scattering with the ambient electrons, which alter the neutrino oscillation patterns. Since the matter effects depend upon the density of electrons, it can be used to shed light on the internal structure of Earth. DeepCore, a densely instrumented sub-array of the IceCube neutrino observatory at the South Pole, detects atmospheric neutrinos over a wide range of baselines with energies as low as about 3 to 5 GeV. We show that this low-energy threshold, access to multiple baselines, high statistics in various oscillation channels, optimized binning scheme in reconstructed energy and zenith, and an efficient particle identification enable DeepCore to observe the presence of Earth's matter effects in three-flavor neutrino oscillations. We further demonstrate that these matter effects in oscillations of atmospheric neutrinos can be used to establish the layered structure inside Earth and measure the mass of Earth and mass of core.
Orateur: Prof. Sanjib Kumar Agarwalla (Institute of Physics, Bhubneswar, India & University of Wisconsin-Madison, USA) -
17:30
Discussion time 30m
-
14:00
-
19:30
→
22:30
Social Dinner 3h Amphitheater Pierre-Gilles de Gennes
Amphitheater Pierre-Gilles de Gennes
APC-Université Paris Cité
-
09:00
→
12:30
-
-
09:00
→
12:15
Mantle-crust connection, geoneutrinos and Earth's heat budget Amphitheater Pierre-Gilles de Gennes
Amphitheater Pierre-Gilles de Gennes
APC-Université Paris Cité
Président de session: Nobuaki Fuji (Université Paris Cité, Institut de physique du globe de Paris)-
09:00
Revealing the Earth’s interior with geoneutrinos 35m
K, Th, and U, with their relative high abundances and half-lives comparable to or greater than the age of the Earth, contribute to approximately the 99% of the radiogenic heat production of our planet. During a beta minus decay, geoneutrinos and radiogenic heat are released in a well-established proportion: measuring the detectable U and Th geoneutrino flux at surface hence translates in providing valuable insights on many open questions about the Earth's interior. By analyzing the energy spectra, one can limit the overall quantity and distribution of U and Th and test different compositional models of the Earth. Additionally, by accurately predicting the geoneutrino signal from the accessible lithosphere, the unknown parameter of the mantle component can be determined, extracting unique information about the radiogenic power and composition of the Earth. The impacts of the recent results from KamLAND and Borexino as well as the expected outcomes from SNO+ and JUNO will be presented.
Orateur: Dr Virginia Strati (University of Ferrara & INFN Ferrara) -
09:35
Overview of experimental results on geoneutrinos 35m
Thanks to the progress in neutrino-detection techniques, geoneutrinos, antineutrinos from the decays of long-lived radioactive elements inside the Earth, can be detected and exploited as a unique tool to study our planet. Geoneutrinos from the 238U and 232Th radioactive chains with energies above 1.8 MeV were measured by the KamLAND experiment in Japan and the Borexino experiment in Italy, exploiting the charge-current inverse-beta decay interaction on protons. Both detectors are located underground and feature large volume liquid scintillator target. The most relevant backgrounds to geoneutrino measurement are represented by reactor antineutrinos, residual muon flux, and intrinsic radioactivity of the detector. Both experiments reached similar precision of the geoneutrino signal measurement in the range of 15 to 18% and confirmed a general consistency of the measured signal with the geological expectations. Due to the different geological settings and geographical locations, their results are complementary. Assuming a laterally homogeneous mantle, their measurements feature some level of tension: Borexino prefers geological models predicting higher U and Th abundances, while KamLAND favors their lower abundances. The talk will describe the key elements of the analysis, overview of the latest results and their geological interpretation, in terms of the corresponding radiogenic heat, geoneutrino signal from the Earth's mantle, and the limit to the hypothetical georeactor.
Orateur: Prof. Livia Ludhova (IKP-2 Forschungszentrum Juelich) -
10:10
Coffee break 30m
-
10:40
Perspectives and challenges for geoneutrino science 40m
In the coming years, the geoneutrino experimental dataset will be enriched beyond the existing Borexino and KamLAND experiments. Data from the Canadian SNO+ experiment are expected very soon, and the construction of the Jiangmen Underground Neutrino Observatory (JUNO) is nearing completion. We are entering an era of multi-site geoneutrino detection.
The road ahead for geoneutrino science is full of opportunities, not only for experimental physicists, but especially for Earth scientists. The geoscience community will play a crucial role in this scientific endeavor by constructing crustal and mantle models to improve the understanding of direct geoneutrino measurements.
Looking even further, the distinctive features of the China Jinping Underground Laboratory and of the pioneering Ocean Bottom Detector promise to refine our understanding of the geoneutrino signals originating from uranium and thorium in the crust and mantle.
The talk will review all these perspectives and challenges for geoneutrino science including the detection of potassium geoneutrinos.Orateur: Prof. Fabio Mantovani (University of Ferrara & INFN) -
11:20
JUNO's Perspective for Geoneutrinos 25m
Jiangmen Underground Neutrino Observatory (JUNO) is a neutrino experiment being built in Southern China to measure neutrinos produced in nuclear power plants at a distance of 52.5 km. Having the main goal to improve the knowledge about neutrino oscillations, fundamental properties of these particles, JUNO will also be able to observe neutrinos of natural origin such as from the Sun, supernovae, the Earth atmosphere and its interior. The latter are called geoneutrinos and can serve as a proxy for investigation of the Earth’s radiogenic heat. Using inverse beta-decay as the detection channel, JUNO is sensitive to geoneutrinos produced in beta-decays of U-238 and Th-232 radioactive isotopes. JUNO will collect in one year about 400 geoneutrinos, what is more than the present-day statistics measured by Borexino and KamLAND together. This talk will report the JUNO expected sensitivity to geoneutrino measurement including some preliminary results of an updated analysis.
Orateur: Dr Yury Malyshkin (GSI / Forschungszentrum Jülich) -
11:45
Discussion time 25m
-
09:00
-
12:15
→
13:30
Lunch 1h 15m Amphitheater Pierre-Gilles de Gennes
Amphitheater Pierre-Gilles de Gennes
APC-Université Paris Cité
-
13:30
→
17:55
Open questions in the study of Earth's mantle and core Amphitheater Pierre-Gilles de Gennes
Amphitheater Pierre-Gilles de Gennes
APC-Université Paris Cité
Président de session: Joao Coelho (APC / CNRS)-
13:30
Geodynamics of core-mantle interactions 25m
(REMOTE)
The present day structure of the core-mantle boundary (CMB) region reflects the cumulative effects of 4.5 billion years of dynamical evolution, shaped by processes in both the core and mantle, which are likely to have experienced major transitions through Earth’s history. Seismologically detected features such as a buoyant alloy-enriched layer floating atop the core, thin (~10 km) ultralow-velocity zones (ULVZ) scattered around the CMB, the two large (~1,000 km) low shear velocity provinces (LLSVP) beneath Africa and the Pacific, seismic discontinuities and scatterers in the lowermost mantle, need to be understood along with mineral physics constraints on the behavior of materials at CMB conditions, geodetic data, geomagnetic secular variations reflecting flows in the shallow core, geochemical analyses of lavas possibly derived from rocks that once circulated at the CMB, cosmochemical and astrophysical constraints on the assembly of planets/moons in the early solar system, tectonic, chemical, isotopic, and magnetic history preserved in the Earth’s lithosphere and crust, and now promising new constraints provided by geoneutrino detectors. In this talk I will focus on integrated models and the ways in which they may influence the concentration and distribution of geoneutrino producing regions in the deep mantle, as well as the over all thermal evolution and energy budget of the Earth.
Orateur: Prof. John Hernlund (Tokyo Institute of Technology) -
13:55
Seismology of core-mantle boundary 25m
The lower mantle of the Earth is home to a variety of anomalous structures with different scales. This includes two large-scale, continent-sized structures called large low shear velocity provinces which are consistently observed across the existing three-dimensional tomographic models. Conversely, small- to meso-scale structures such as a ultralow-velocity zone (ULVZ) or mega-ULVZ are often beyond the current resolution of those tomographic models but studied by using seismic wavefield simulations with various seismic phases which have been scattered or multipathed by such heterogeneities in the core-mantle boundary region. Although the origin and nature of these structures are still largely unknown, recent studies suggest that they are capable of hosting primordial geochemical reservoirs and are therefore intimately tied to important geological processes and evolution that entail interactions between contrasting materials for the mantle and core, possibly aided by deep-seated mantle plumes. In my presentation, I will provide a brief summary of seismological constraints pertaining to the lower mantle structures of the Earth.
Orateur: Dr Doyeon Kim (ETH Zurich) -
14:20
Probing the properties of Core-Mantle Boundary using Atmospheric Neutrino Oscillation 20m
(REMOTE)
Information about the interior of Earth is obtained from indirect probes used in traditional seismic and gravitational studies. While gravitational measurements give precise values of the mass and moment of inertia of Earth, seismic measurements tell us about the physical and chemical properties of Earth. Complementary information may be obtained using the observations of neutrinos that pass through Earth. While propagating through the different regions inside Earth, the multi-GeV energy range neutrinos encounter the Earth’s matter effects due to the forward elastic scattering with the ambient electrons, which alter the neutrino oscillation probabilities. These matter effects depend upon the energy of neutrinos as well as the density distribution of electrons they encounter during their propagation.
In this talk, we present how well an atmospheric neutrino oscillation experiment like Iron Calorimeter (ICAL) detector at India-based Neutrino Observatory (INO) would probe the location of the core-mantle boundary (CMB) and the density jump at CMB. The ICAL detector with 1000 kt·yr exposure would be able to locate the CMB with a precision of about ±250 km and probe the density jump at CMB with a precision of about 15% at the 1σ confidence level. We demonstrate that the charge identification capability of ICAL would play an important role in achieving these precisions.Orateur: Anuj Kumar Upadhyay (Aligarh Muslim University, Aligarh & Institute of Physics, Bhubneswar, India) -
14:40
Probing interiors of Earth using magnetized neutrino detector 25m
Atmospheric neutrinos offer the possibility of exploring the internal structure of Earth. This information is complementary to the traditional seismic and gravitational studies. While propagating through Earth, the upward-going multi-GeV neutrinos encounter the so-called Earth’s matter effects due to their coherent forward scattering with the ambient electrons, which alters the neutrino oscillation probabilities significantly. The dependence of matter effects on the electron density of medium can be used to probe the internal structure of Earth. In this talk, I will discuss how well an atmospheric neutrino oscillation experiment like the proposed 50 kt magnetized Iron Calorimeter (ICAL) detector at the India-based Neutrino Observatory (INO) would validate the presence of Earth’s core, measure the location of the core-mantle boundary (CMB), and probe the possible presence of dark matter inside the Earth in a unique way through matter effects in neutrino oscillations deep inside the Earth. ICAL can perform these studies owing to its good angular resolution, which helps in observing the core-passing neutrinos efficiently. Also, due to its magnetized setup, it would be able to observe neutrinos and antineutrinos separately, which in turn plays an important role to study different matter effects in neutrino and antineutrino modes.
Orateur: Dr Anil Kumar (DESY, Zeuthen, Germany) -
15:05
Coffee break 30m
-
15:35
Earth tomography with supernova neutrinos and the first neutrino tomography of Earth 40m
There are two approaches to perform Earth tomography with neutrinos: (i) neutrino oscillation tomography, based on coherent Earth matter effects modifying neutrino oscillations patterns and (ii) neutrino absorption tomography, based on partial absorption of a neutrino flux as it propagates through the Earth. Oscillation tomography is usually discussed in the context of GeV atmospheric neutrinos. In the first part of this talk, however, I will focus on supernova neutrinos with tens of MeV. Whereas at GeV energies, Earth matter effects are driven by the atmospheric mass-squared difference, at energies below ∼ 100 MeV, it is the solar mass-squared difference what controls them. The capabilities of future neutrino detectors, such as DUNE, Hyper-Kamiokande and JUNO will be presented, including the impact of the energy resolution and other factors. In the last part of this talk, I will present the first, and so far only, neutrino tomography of Earth with actual data. This was performed with IceCube data, by studying the absorption of atmospheric neutrinos after crossing the Earth.
Orateur: Dr SERGIO PALOMARES RUIZ (IFIC-CSIC) -
16:15
Testing Neutrino Oscillation Tomography as a tool for sensing LLSVPs density 20m
Global seismic data reveals the existence of two, continent-sized anomalous regions beneath Africa and the Pacific Ocean. These regions are defined by lower than average seismic shear wave speed hence the name of Large Low Shear Velocity Provinces (LLSVPs). LLSVPs cover ~20% of the Core Mantle Boundary (CMB) and extend into the lowermost mantle up to 1000 km above the core-mantle boundary. LLSVP are hypothesized to be either thermal anomalies or a combination of both thermal and chemical anomalies in the lower mantle. Observations find an anticorrelation between shear wave and bulk sound speed that points toward the thermochemical hypothesis. If LLSVPs are indeed thermochemical anomalies, the exact nature of the compositional component and particularly its density is debated. Recent studies disagree when predicting whether LLSVPs are more or less dense than the surrounding mantle. For example, Tidal Tomography suggests a denser composition (~1 ± 0.5%) and analysis of Stoneley wave data suggest a less dense composition. These disparities demonstrate the need for further constraints on LLSVP density and recommend the exploration of novel techniques. Here, we explore Neutrino Oscillation Tomography as an alternative methodology to constrain LLSVPs density
For this work we used a custom code that implements the package OscProb to simulate the evolution of atmospheric muon neutrinos through the Earth’s lower mantle and their corresponding signal at a simulated detector located at the Earth’s South Pole. We present the distribution in energy and direction of observed events of a Water/Ice Cherenkov detector for two models of the Lower Mantle: 1) a standard earth defined by the PREM model and 2) a Lower Mantle with a single LLSVPs (defined as a slab of 400 km height and 4000 km lateral extension of material that is either 1% denser or 1% less dense that the background mantle)
Orateur: Yael Armando Deniz Hernandez (University of Idaho) -
16:35
DUNE perspectives for atmospheric neutrino detection and studies 20m
(REMOTE)
The Deep Underground Neutrino Experiment (DUNE) is a flagship neutrino oscillation experiment to be built in the US and operated by a large international collaboration. The DUNE setup is primarily designed as a long-baseline accelerator neutrino experiment to make precise measurements of neutrino oscillations. The DUNE Far Detector (FD) will be constructed as four separate Liquid Argon Time Projection Chamber (LArTPC), with a total LAr mass of 70 kt. It will be placed underground with a rock cover of 1500 m at the Sanford Underground Research Facility, South Dakota, greatly lowering muon background. The LArTPC modules will allow for very high spatial resolution for particle tracking in neutrino interactions, promising excellent neutrino energy and direction reconstruction. These characteristics will enable the DUNE FD to function as an excellent atmospheric neutrino detector. In this contribution, we present some important features and the physics potential of atmospheric neutrino measurements in the DUNE FD.Orateur: Dr Tarak Thakore -
16:55
Earth shaped by primordial H2 atmospheres 30m
Earth's water, intrinsic oxidation state, and metal core density are fundamental chemical features of our planet. Studies of exoplanets suggest that hydrogen-rich atmospheres may be the source of these features. Planet formation and evolution models demonstrate that rocky exoplanets commonly formed with hydrogen-rich envelopes that were lost over time. These findings suggest that Earth may also have formed from bodies with H$_2$-rich primary atmospheres. We have applied a self-consistent equilibrium chemical thermodynamic model to show that Earth's water, core density, and overall oxidation state can all be sourced to reactions between H$_2$-rich primary atmospheres and underlying magma oceans in its progenitor planetary embryos. Water is produced from dry starting materials resembling enstatite chondrites as oxygen from magma oceans reacts with hydrogen. Hydrogen derived from the atmosphere enters the magma ocean and eventually the liquid metal core. The resulting ~ 0.5 wt % H in the core is the primary source of density deficits matching that of Earth's core. Oxidation of the silicate rocks from solar-like to Earth-like oxygen fugacities also ensues as Si, along with H and O, alloys with Fe in the cores, contributing further to the core density deficits. Reaction with hydrogen atmospheres and metal-silicate equilibrium thus provides a simple explanation for fundamental features of Earth's geochemistry that is consistent with rocky planet formation across the galaxy.
Orateur: Prof. Edward Young (University of California Los Angeles) -
17:25
Discussion time 30m
-
13:30
-
17:55
→
19:30
Wine and Cheese 1h 35m Amphitheater Pierre-Gilles de Gennes
Amphitheater Pierre-Gilles de Gennes
APC-Université Paris Cité
-
09:00
→
12:15
-
-
12:30
→
14:00
Lunch 1h 30m Amphitheater Pierre-Gilles de Gennes
Amphitheater Pierre-Gilles de Gennes
APC-Université Paris Cité
-
14:00
→
15:00
Summary of the workshop Amphitheater Pierre-Gilles de Gennes
Amphitheater Pierre-Gilles de Gennes
APC-Université Paris Cité
-
14:00
Summary: geosciences point of view 30mOrateur: Prof. Nobuaki Fuji (IPGP)
-
14:30
Summary: neutrino point of view 30mOrateur: Prof. Véronique Van Elewyck (APC)
-
14:00
-
15:00
→
15:30
White paper discussion amphitheater Pierre-Gilles de Gennes (APC - Université Paris Cité)
amphitheater Pierre-Gilles de Gennes
APC - Université Paris Cité
-
15:30
→
16:00
Coffee break 30m Amphitheater Pierre-Gilles de Gennes
Amphitheater Pierre-Gilles de Gennes
APC-Université Paris Cité
-
16:00
→
17:00
White paper discussion amphitheatre Pierre-Gilles de Gennes (APC - Université Paris Cité)
amphitheatre Pierre-Gilles de Gennes
APC - Université Paris Cité
-
12:30
→
14:00